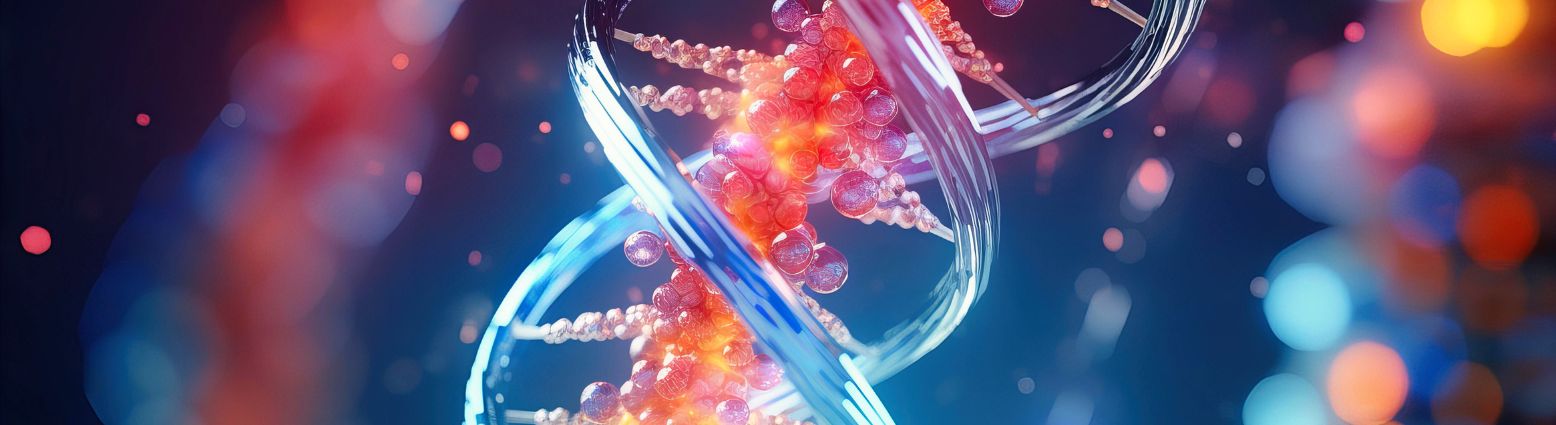
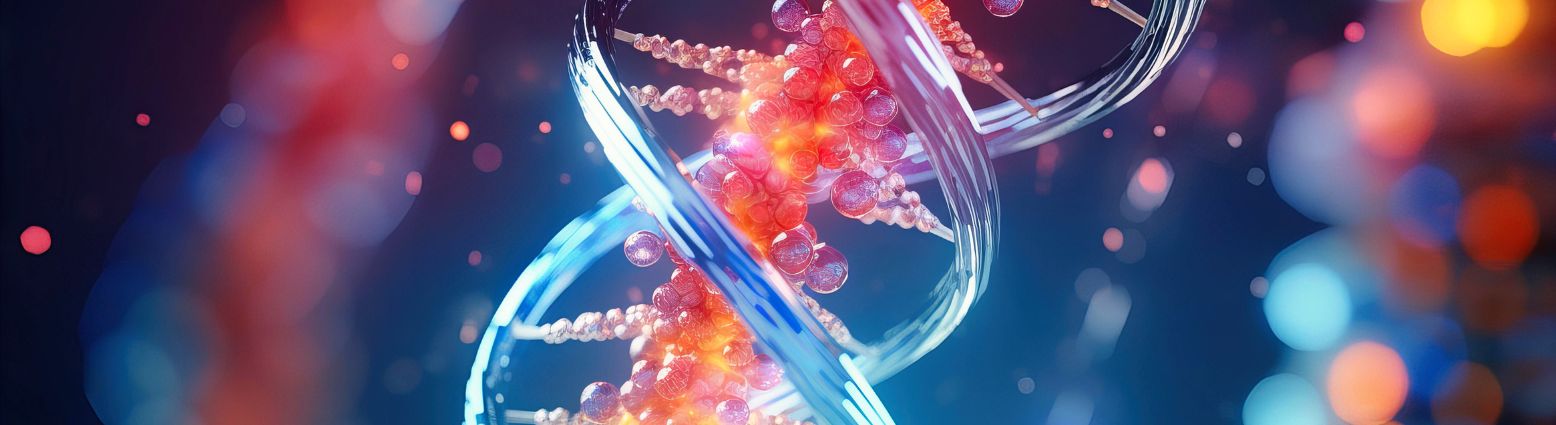
Small Molecule Strategies for RNA-Targeted Drug Discovery Molecular approaches and Improved cell-based assays for RNA target drug discovery
Overview on RNA-targeted compounds
What is targeted RNA Drug Discovery?
The complex and dynamic3D structure of RNA plays a pivotal role in human biology and contributes to disease pathology. Efforts to target these RNA structures therapeutically with small molecules, although nascent, are advancing rapidly. Key progress includes the refinement of computational tools for predicting conserved RNA structures and the implementation of strategies to broaden therapeutic scope.
Current small molecule interventions employ diverse mechanisms, including directing splicing (e.g., Branaplam and FDA-approved Risdiplam), inhibiting translation of challenging proteins, and deactivating functional structures in noncoding RNAs.
RNA Structure-Targeted Drug Discovery services
There are different methods and strategies to identify small-molecule RNA binders in Drug Discovery:
Targeting RNA with Small Molecules
Traditionally, drug discovery efforts have primarily focused on targeting proteins, but there is growing interest in developing small molecules that interact with RNA molecules. These molecules can modulate RNA function by altering RNA folding, stability or interactions with other molecules.
RNA Structure and Function
RNA molecules can adopt complex three-dimensional structures that play critical roles in cellular processes such as gene expression and protein synthesis. Small molecules can be designed to specifically recognize and bind to these RNA structures, thereby modulating their function.
Screening Approaches
High-throughput screening (HTS), fragment-based screen and in silico computational methods are commonly used in the discovery of small molecule binders of RNA. HTS involves testing large libraries of small molecules to identify compounds that exhibit binding affinity and specificity for the target RNA. Computational methods, such as molecular docking and molecular dynamics simulations, can help in predicting the binding modes and affinity of small molecules to RNA targets.
RNA Target Classes
RNA molecules targeted for drug discovery can include messenger RNA (mRNA), non-coding RNAs (such as microRNAs and long non-coding RNAs), ribosomal RNA (rRNA), transfer RNA (tRNA) and viral RNA. Each RNA target class presents unique challenges and opportunities for small molecule drug discovery.
Therapeutic Applications
Small molecule binders of RNA have the potential to be developed into therapeutics for a wide range of diseases. For example, small molecules that target viral RNA can inhibit viral replication and are being explored as antiviral agents. Similarly, small molecules that modulate the function of disease-associated non-coding RNAs are being investigated as potential treatments for cancer and other disorders.
Traditionally, drug discovery efforts have primarily focused on targeting proteins, but there is growing interest in developing small molecules that interact with RNA molecules. These molecules can modulate RNA function by altering RNA folding, stability or interactions with other molecules.
RNA molecules can adopt complex three-dimensional structures that play critical roles in cellular processes such as gene expression and protein synthesis. Small molecules can be designed to specifically recognize and bind to these RNA structures, thereby modulating their function.
High-throughput screening (HTS), fragment-based screen and in silico computational methods are commonly used in the discovery of small molecule binders of RNA. HTS involves testing large libraries of small molecules to identify compounds that exhibit binding affinity and specificity for the target RNA. Computational methods, such as molecular docking and molecular dynamics simulations, can help in predicting the binding modes and affinity of small molecules to RNA targets.
RNA molecules targeted for drug discovery can include messenger RNA (mRNA), non-coding RNAs (such as microRNAs and long non-coding RNAs), ribosomal RNA (rRNA), transfer RNA (tRNA) and viral RNA. Each RNA target class presents unique challenges and opportunities for small molecule drug discovery.
Small molecule binders of RNA have the potential to be developed into therapeutics for a wide range of diseases. For example, small molecules that target viral RNA can inhibit viral replication and are being explored as antiviral agents. Similarly, small molecules that modulate the function of disease-associated non-coding RNAs are being investigated as potential treatments for cancer and other disorders.
Oncodesign Services & Zobio : a solid alliance of partners to provide experimented services for RNA-targeted development
Oncodesign Services is a leading CRO specialized in Drug Development and Preclinical Services. In 2024, Oncodesign Services made the acquisition of Zobio, a class-leading platform dedicated to small molecule discovery. The 2 partners provide a fully integrated offering to support innovative programs from target validation to candidate selection.
Phenotypic Screening in Small Molecule Drug Discovery Targeting RNA
In the context of small molecule RNA binder discovery, phenotypic screening is a valuable approach that involves evaluating the effects of small molecules on cellular or organismal phenotypes without prior knowledge of the target.
- Broad Discovery Potential: Phenotypic screens offer a way to identify small molecules that modulate RNA function without prior assumptions about the specific target or mechanism of action. This approach can uncover unexpected connections between small molecules and RNA targets, leading to the discovery of novel therapeutic candidates.
- Capturing Complex Interactions: RNA molecules often interact with multiple proteins and cellular components to exert their biological functions.
The chosen cells may be engineered, if necessary, either to mimic the desired ‘disease’ state or to enable high-throughput screening.
Developing and conducting phenotypic screens throught various methods
Target Agnostic Approach: Unlike target-based screens, phenotypic screens do not require prior knowledge of the specific RNA target involved in disease pathology. This is particularly advantageous when studying complex RNA regulatory networks or when the target is unknown or poorly characterized.
Example of a target agnostic approach
Target-based phenotypic screen: Cell lines can be precisely genetically manipulated to align with a desired phenotype. This genetic engineering process enables the development of tailored cell lines for High Throughput Screening (HTS) protocols, facilitating efficient and accurate screening strategies for identifying compounds with specific therapeutic effects.
Providing Biophysical methods to discover or characterize RNA hit binders
Biophysical methods play a unique role in target-based drug discovery, enabling detailed kinetic, thermodynamic and structural characterization of small-molecule target interactions. These methods are generally label-free and detect direct interaction between small molecules and a target RNA, being suitable both as primary screening approaches and as validation assays.
- Surface Plasmon Resonance (SPR)
SPR is a moderate throughput and direct method to detect binding of small molecules to RNA. In SPR, one binding partner (in this case the target RNA) is immobilized on a functionalized gold surface and the other binding partner is flowed over the surface in solution. Binding interaction results in the refractive index change on the surface which is detected as an SPR response. Using continuous flow microfluidics, the method monitors the interaction of the small molecules injected over the immobilized target in real time. Utilizing a relatively small quantity of RNA, a modern high throughput SPR instrument allows analysis of up to 1,000 interactions per day. Identified hits from the primary screen are further confirmed by titration into the immobilized target RNA for affinity determination. In addition to be label-free, the primary advantage of SPR is its ability to measure both the affinity (KD) and the kinetics (ka and kd) of binding in real time (Figure 1). The affinity and kinetic parameters are valuable information for medicinal chemists to design optimized compounds with improved drug-like properties.
Figure 1: Monitoring the interaction of Paromomycin binding to an immobilized bacterial S16 Ribosomal RNA. A) Biotinylated Helix 44 of bacterial S16 Ribosomal RNA was immobilized on a streptavidin coated gold sensorchip. An increasing concentration of Paromomycin was injected over the surface. B) The interaction of paromomycin with the rRNA resulted in an SPR response which was monitored over time (colored traces). The resulting SPR traces are called sensorgrams and can be fit to a kinetic model (black traces) to determine the affinity and kinetics of binding.
- NMR spectroscopy
NMR spectroscopy is a low-throughput, but powerful, solution-based technique that can be used to monitor RNA – small-molecule interactions (both weak and tight), to map binding sites, to assess RNA folding, to determine high-resolution structures and to characterize conformational dynamics.
RNA – small-molecule interactions are readily identified in simple 1D 1H spectra that can either focus on the ligand signals (ligand-observed NMR) or on the RNA signals (RNA-observed NMR). The latter will also provide low-resolution information on the folding and integrity of the RNA molecule (Figure 2).
Figure 2: 1D 1H spectrum of HIV-1TAR stem loop (29 nt). Typical 1H chemical shifts for different groups are marked. The distinct chemical shift of imino protons involved in Watson-Crick base pairs provides information on folding.
Making use of 2D and 3D NMR experiments and labeling with stable isotopes (13C and 15N), the resonances (peaks) of RNA spectra can be assigned, unlocking increasingly detailed analyses: the binding site of small-molecules of interest can be mapped, the effect of ligands on local conformations can be assessed and high-resolution structures of the complexes can be determined (Figure 3).
Figure 3: Binding site mapping of acetylpromazine. (left) Titration of HIV-1 TAR stem loop (29 nt) with acetylpromazine : 2D 1H, 1H TOCSY spectra of HIV-1 TAR in the presence of increasing amounts of acetylpromazine. (right) The bases that show chemical shift perturbations upon addition of ligand are highlighted in blue on the structure of HIV-1 TAR, delineating the binding site for acetylpromazine.